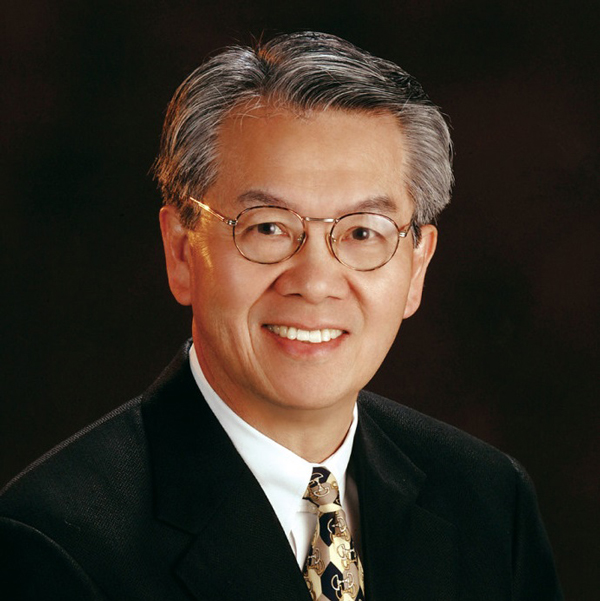
Address: No.250, Wu Hsing Street, Taipei City 110, Taiwan (R.O.C.)
Telephone: +886 02-27361661 ext.8902
E-mail: tmutirc@tmu.edu.tw
© Copyright – TMU Translational Imaging Research Center – design by Morcept
Professor Cheng-Yu Chen took over as director of Imaging Research Center (IRC) in March 2014. In order to initiate and promote the academic research, clinical service and human capacity building, the amendment of University Affairs Meeting had been passed in October 2014 for proposing a higher level of research center named Translational Imaging Research Center (TIRC) on attachment to the medical college at Taipei Medical University. Subsequently, TIRC headed toward studies of translational imaging by increasing the quantity and quality of research instruments and research fellows.
With respect to facilities for small animal imaging research, the 7T MRI had been installed and passed the safety test in July 2015. The 3T MRI for clinical research would be installed in Taipei Medical University Hospital, and core laboratories in TIRC have been setting up actively for integration with related research fields such as genetic and cellular studies, animal model of diseases, and clinical trials, which may lead to Industry-Academia cooperation as one of the future goals. Imaging Research Center has renamed formally as Translational Imaging Center (TIRC) since May 2015, committing with a macroscopic view to devoting and promoting research issues more vividly and characteristically as an academic research center with international perspective.
TIRC has built multiple image platforms for animal experiments and clinical trials in an effort to promote medical imaging research, including establishment of various animal models of human disease in comparison with findings of physiological and pathological changes from clinical medicine images. Via the delicate instruments and precisely designed laboratory animal models combined with imaging studies and staining techniques, TIRC may also help medical physicians to clarify the clinical pathology and disease diagnosis by providing evidence-based imaging results.
Meanwhile, TIRC observes the various mechanisms of neuro-signal pathways on disease regulation and pharmacology through experiments in genetics, cell biology and molecular biology. As for clinical trials, the psychophysiological assessment may serve as an important prognostic indicator of diseases by reflecting the mental state and social function of patients after the onset of an illness.
The goal of research is to develop more effective medical image markers for early diagnosis and prognosis evaluation of diseases. By utilizing the non-invasive quantitative model of medical imaging, we may build an effective screening platform used for evaluating drug efficacy and therapeutic effects. The field of research involves genetic and cellular studies, laboratory animal experiments, and research studies on human participants. The advanced technique of translational medical imaging platform is beneficial for effective biomarkers, R&D of new drugs, and translational medicine research. Apart from technical development of medical imaging, its application would support the diagnosis and treatment of four main neurological diseases.
The central aim is to integrate relevant research teams in medical imaging at Taipei Medical University, establish advanced imaging facilities, provide consultation for both animal and human imaging research, develop advanced imaging and analysis techniques, and promote medical imaging while nurturing talent.
The research and development focus on translational imaging research in neurological disorders, leveraging the diverse expertise of center members. Five core laboratories have been established:
These labs span translational imaging research across different levels, from genes, cells, and animals to human studies.
The center aims to foster a comprehensive research chain, conceptualizing the “translation” from “bench-bed” to “bed-industry”.
Current research areas include:
Brain Tumor
Glioblastoma Radiogenomics: The research topic is mainly focused on glioblastoma multiforme(GBM). We will investigate the heterogeneity of MR imaging findings between inter- and intra-tumors, and also explore the correlation between MR imaging findings and gene mutations. We have glioblastoma cell culture with specific genetic information, such as tumor formation, angiogenesis, gene clusters associated with DNA damage and repair. Furthermore, an animal model of glioblastoma multiforme will be established at 7T MRI, and the same method can be applied to MR imaging study on human participants at 3T MRI.
Meanwhile, the information of gene mutation in GBM can be obtained from DNA microarray experiment, and the molecular expressions of glioblastoma multiforme with different genomic modifications are compared with MRI-based biomarkers. With the combined application of genomic information and advanced multiparametric MRI, the tumor extent can be accurately defined, the therapeutic response can be better monitored and the prognosis can be well predicted. In brief, this bedside-to-bench translational research in TIRC is aimed to establish a radiogenomics platform based on advanced MR imaging for the purpose of understanding the genomic modification of glioblastoma multiforme and its relation with the perspective of molecular MRI.
The interaction of glioblastoma and drug therapy, the development of prognostic factors in imaging: Recent studies have been investigating and monitoring the progression of tumors with molecular MRI. Particularly, the non-invasive magnetic resonance imaging (MRI) is a mature technology for diagnosing brain tumors as it offers detailed images with high resolution that are provided by combination of contrast agents and antitumor agents. We assume that the therapeutic response in GBM (includes drug resistance, the localized recurrence rate, etc.) would be affected by Temozolomide (TMZ) induced activation in neural pathways within the brain due to its modification to signals of DNA sequence in tumor cells.
We indicate the content of DNA repair signaling pathways within the tumor cell via the advanced magnetic resonance imaging biomarkers, which a multi-parametric imaging approach is taken. The well-established knowledge of the relation between imaging biomarkers and TMZ-based therapeutic reaction may contribute meaningfully to future diagnosis of GBM.
Bevacizumab (Avastin) is a drug as angiogenesis inhibitor for cancer therapy. It slows the growth of new blood vessels and decreases the volume of tumor cells by inhibiting vascular endothelial growth factor A (VEGF-A), and is usually combined with target-therapy for treating brain tumors. Examining the volume of tumor by using MRI is one of the methods to evaluate the therapeutic reaction of Bevacizumab, which mainly uses the contrast agent to enhance the image of blood-brain barrier within the tumor.
However, some of the drugs for treating glioblastoma multiforme may lead to misdiagnosis due to its false repair of blood-brain barrier within the brain. So far there have been seldom appropriate MRI methods to avoid the false repair and to illustrate the region of tumors within brain. We aim to use molecular nanotechnology combined with both MRI and Bevacizumab to establish a platform for diagnosis and treatment of GBM based on MRI images.
In conclusion, based on the research of radiogenomics of GBM, TIRC carries out the core ideology of translational imaging research as with NMR and BER pathways of tumor cell chemoresistance mechanisms, TMZ-resistance mechanisms based on animal models of GBM, innovative MRI technology and quantitative imaging biomarkers development, and radiogenomic analysis of GBM. The goal of research is to explore and estimate TMZ-resistance mechanisms of GBM relied on MRI methods. Clinical trials of drugs (MPT0B291, SP1 and mTOR inhibitors) combined with above research findings will be conducted as future applications.
Ischemic Stroke
Ischemic Stroke Multiparametric MR imaging analysis of ischemic stroke:
The therapeutic effect of treatment in ischemic stroke relies not only on the recovery of blood flow, but also the amount of possibly non-impaired neurons in the human brain after stroke. Within 4.5 hours after the cerebrovascular accident is usually a critical and valuable period of time to give treatments. Therefore, defining the onset time of CVA, locating the lesion sites as well as evaluating the preserved brain tissues precisely are all important factors to consider before any intervention or thrombolytic drugs injection are given.
There have been many MR imaging related studies in ischemic penumbra, which proposed the ischemic penumbra could be referred as the location of possibly preserved neurons. Furthermore, its area and size may be regarded as a predictor for therapeutic efficacy of pharmalogical treatments. Generally, both diffusion tensor imaging (DTI) and perfusion-weighted imaging (PWI) are advanced MRI techniques essentially for evaluating ischemic penumbra, yet the contrast agent used in PWI is not suitable for patients with certain conditions.
The result of our research illustrated that the multiple parameters calculated from DTI could be treated as an effective indicator for assessing the region of ischemic penumbra and estimating the onset time of ischemic stroke. It had been tested and verified in a stroke model of rats. Currently the application of laboratory research findings under the advanced MRI method has already been linked to studies involving human participants. The high availability of DTI will provide clinicians a new opportunity for diagnosis and treatment of ischemic stroke.
Secondary trans-neuronal degeneration after stroke:
Transneuronal degeneration (TND) is thought to be linked with cerebral vascular accident (CVA), which commonly lasts for several days to weeks from the acute stage of injury. It might occur in the intact brain regions involved in motor function such as the motor pathways or the striatonigral pathway in the extrapyramidal system, which causes the so-called post stroke movement disorders.
The long-term impairments in motor skills, memory function and consciousness disturbance result from TND are unpredictable, negatively impacting the arrangement of rehabilitation and the quality of life in patients with a poor outcome of disease progression. TIRC has created detailed images of the brain structure and function measured by advanced MR imaging techniques (e.g., diffusion tensor imaging, q-space imaging, resting-state functional MRI, cerebral perfusion MRI, susceptibility weighted imaging, and magnetic resonance spectroscopy).
The purpose of the ongoing research projects is to investigate the pathological mechanisms of post-stroke TND, which aims at exploring the relation between TND and the networks of cortical-subcortical regions. The prominent findings of laboratory animal studies will be applied to stroke-related clinical researches. A key goal is to enable more precise identification of biomarkers relying on medical imaging, and to enhance the ability of early detection of brain-related diseases with reliable biomarkers.
The Functional Connectivity of Thalamic Networks after Acute Stroke:
The secondary trans-neuronal degeneration (TND) usually happens as a post-stroke symptom. The thalamus is generally recognized as a relay between cortical and subcortical networks. It plays an important role in processing sensory information (e.g., the ventral posterior thalamic nucleus and the ventral posterolateral thalamic nucleus), coordinating motor function (e.g., the ventral anterior nucleus and the ventral nucleus), and regulating states of consciousness.
The anterior nuclei of thalamus is associated with memory function. Despite blood supply for the thalamus largely comes from the basilar artery and the posterior communicating artery, other specified brain lesions will lead to the secondary TND of thalamus due to its connection with the cerebral cortex. There have been studies concerning the impairment of thalamus result from the secondary TND of infarction or ischemia in the territory supplied by the middle cerebral artery (MCA). However, the alternation of thalamic nucleus and the circuitries/functional connectivity between thalamus and the cerebral cortex have seldom been investigated.
Questions about the relation of TND and thalamic nucleus, the clinical symptoms found in the interaction between lesions of thalamic nucleus, and whether or not the apoptosis across a synapse could be prevented have all not been defined well yet. TIRC’s research groups aim to study, analyze and compare the stroke model of laboratory animals with the research of CVA patients. The goal of research will emphatically focus on the mechanism of the secondary TND in thalamus.
First of all, a parcellation scheme with respect to the specific localization of thalamic nucleus will be carried out using structural MRI. Secondly, based on the parcellation of thalamic nucleus, we may characterize the functional anatomy of thalamus by using advanced MR imaging techniques, such as diffusion tensor imaging, q-space imaging, resting-state functional MRI, cerebral perfusion MRI, susceptibility weighted imaging, and magnetic resonance spectroscopy.
Finally, in order to explore the pathological mechanism of TND following a cardiovascular accident, the research theme will focus on specific regions of the cerebral cortex. The photothrombotic stroke model of rats is applied to research of correlation between thalamocortical circuit and TND. Meanwhile, the MR imaging findings of TND in rats will link with the results of pathological data, which is ultimately applied to stroke-related clinical researches.
The research hypothesis is proposed that the pathological oscillation within thalamus induced by the secondary TND is specifically correlated with the atypical function of thalamocortical pathways. The longitudinal follow-up of TND induced change in the brain will be carried out, and the information analyzed from temporal characteristics of dynamic functional connectivity may be offered to better understand the effects of stroke, as well to give diagnosis and treatment more accurately. Finally, the goal of research is to enable more precise identification of biomarkers relying on medical imaging, and to enhance the ability of early detection of brain-related diseases with reliable biomarkers.
Applying brain compliance to investigate the pressure of cerebral venous:
Brain compliance was initially used to indicate the state of brain homeostasis, which could be affected by intracranial pressure and intracranial volume. The brain homeostasis is maintained normally relying on balance between the intracranial constituents that contains blood, cerebrospinal fluid (CSF), and brain tissue. When brain homeostasis or the state of volume equilibrium is not maintained, the intracranial pressure is elevated with decreased brain compliance. Thus, either the information about mediation of intracranial volume or the risk evaluation of intracranial hypertension can be obtained by measuring brain compliance accurately.
Motion-sensitive magnetic resonance imaging is a non-invasive technique used for monitoring volume arterial inflow, venous blood outflow and CSF during a cardiac cycle. On the other hand, the cine displacement-encoded MRI can be used to record the electric impulses sent by neurons in brain. We aim to explore the underlying mechanism and the correlation between volume arterial inflow, venous blood outflow, CSF and nerve impulse in brain during a cardiac cycle. We will obtain motion-sensitive magnetic resonance imaging from laboratory animals at 7T MRI and from human participants at 3T MRI. We will also propose an algorithm design to improve the estimation of brain compliance, which is expected to offer high value for clinical application in the future.
Mild Traumatic Brain Injury
The relation between thalamocortical dysrhythmia (TCD) and post-concussive syndrome (PCS) in mild traumatic brain injury (mTBI) patients has recently been recognized in several studies; however the potential imaging biomarkers to evaluate neurological functional deficits and to localize TCD, in particular the thalamocortical circuits, have not been established. Based on the current understanding of the mTBI, network dysfunction of thalamocortical circuits is the one of main points of investigation which is characterized by microstructural injuries, disrupted functional connectivity, and the altered interplay between excitatory and inhibitory neurotransmitters. The elevated synaptic glutamate levels after mTBI can further exacerbate the post-traumatic cellular injury. Recent studies have indicated that mTBI patients can benefit from N-acetylcysteine (NAC) treatment by effectively improving the behavioral deficits which had a significant impact on neuropsychological test results.
Though the cognitive tests outcome after administration of NAC is promising, the neuroimaging evidence of NAC treatment efficacy on mTBI and its prevention of subsequent brain atrophy had not been well explored. The purpose of this study is to propose a TCD model in mTBI by simultaneous measurements of MRI and MR-compatible EEG on human participants followed by a pre-clinical experiment using animal mTBI model to evaluate the therapeutic effect of NAC on mTBI. Through the characterization of the neural network dysfunction from the perspectives of neural electrophysiology, microstructure integrity, and hemodynamic synchrony, we will be able to have a complete vision on the pathophysiological mechanism in mTBI. In addition, this study can help provide the neuroimaging evidence of the NAC efficacy in treating mTBI.
Osteoporosis
Osteoporosis is a metabolic bone disease that is featured with low bone mineral density (BMD) and high fracture incidence. Fractures that occur as a result of underlying osteoporosis are significantly associated with elevated mortality risk and loss of disability-adjusted life years. Loss of bone mass is one of critical characteristics of osteoporosis, which is typically diagnosed using BMD measurements. Dual energy X-ray absorptiometry (DXA) method is the most widely used method of assessing BMD. In general, BMD data could be obtained at various skeletal sites such as spine, hip and forearm. Clinically, BMD obtained from lumbar vertebrae could be regarded as major diagnosis dependency for osteoporosis.
Low-dose chest computed tomography (LDCT) is popularly used for early lung cancer screening with less ionizing radiation and has been demonstrated to significantly reduce mortality from lung cancer. LDCT scans generally cover the upper thoracic vertebrae. The covering part of the spine has been suggested to detect patients with osteoporosis. The utility of vertebral CT numbers derived from LDCT for detecting osteoporosis has been confirmed.
On the other hand, CT-based texture analysis appreciates image heterogeneities that may not be discern with the human eye, and preliminary evidence has suggested its potential value in imaging characterization for diagnostic purposes. This method is based on mathematical approaches to the evaluation of gray-level intensity and position of the pixels within the image, providing the so-called “texture features” that represent a quantitative measure of heterogeneity. Patient with osteoporosis may reveal different inherent texture from normal BMD because structural integrity of trabecular bone is impaired. We hypothesized that the bone status could be related to the texture extracted from images. Therefore,we has developed a machine learning-based model based on the texture analysis, which will automatically detect osteoporosis from LDCT scans during lung cancer screening.
Address: No.250, Wu Hsing Street, Taipei City 110, Taiwan (R.O.C.)
Telephone: +886 02-27361661 ext.8902
E-mail: tmutirc@tmu.edu.tw
© Copyright – TMU Translational Imaging Research Center – design by Morcept